Don’t Waste the Waste: Sustainable biomass sourcing for Biochar Carbon Removal
Twenty years ago, waste biomass was literally just that: waste. Today, it is becoming known as a critical resource for the sustainable transformation of our global economy. Reason enough to investigate how biochar carbon removal (BCR) fits into the landscape of biomass use cases. In this article, we explore the requirements for sustainable feedstock sourcing, assess the global availability of unused or unproductively utilized biomass residues, and discuss different applications. Note that we will use the words ‘waste’ and ‘residues’ interchangeably, although in many jurisdictions the classification of a biomass as waste has specific implications regarding its use.
Sustainable sourcing of biomass residue feedstocks
Sustainable sourcing of biomass residues is the very basis for achieving positive environmental additionality with BCR. Taking a holistic impact management perspective, we have defined three sourcing criteria to set the boundaries for our feedstock availability analysis:
- First, BCR should not supersede other uses that can extract more environmental or social value from a biomass.
- Second, pyrolyzing a waste biomass should not lead to higher near-term emissions than if that biomass had been left to decay. Remember that during pyrolysis about 50% of the carbon contained in a substance reacts into CO2 and, by conversion into energy, is ultimately released (unless captured) – whereas in some cases, such as wood, natural decay of a biomass can take many decades until carbon is returned to the atmosphere as CO2.
- And third, potential negative biodiversity impacts of removing waste biomass from an ecosystem, such as habitat destruction or a lack of nutrients in the soil, should be avoided.

Now, it sounds like there might not be much feedstock left for BCR after applying these criteria, doesn't it? No, far from it. In fact, global production of biomass is predicted to increase by 2.5x by 2060 due to demand for food, feed, clothing, other consumer goods, and timber products. The clou: as we grow and extract more virgin biomass to provide for a growing population, the volume of waste byproducts will also increase. These byproducts include forestry residues like sawdust and offcuts, agricultural residues like straw, husks, and manure, and food processing byproducts such as nutshells. Moreover, nature produces unwanted biowaste in the form of invasive plant species: In Namibia, for example, the encroachment of the invasive acacia bush has led to such a threat to open grasslands and wildlife that farmers have started turning it into biochar. And the Sargassum algae has been plaguing coastal ecosystems for many years now.
Large portions of biomass residues are not currently in productive and sustainable use. Take the example of manure: while it can be used for bioenergy and as a fertilizer, in practice significant overproduction results in farmers either sending manure over long distances or overfertilizing their lands. This, in turn, has negative repercussions including increased emissions of methane and nitrous oxide, soil degradation, groundwater pollution, and eutrophication of waterways. A first hint that the ideal use case for biomass may depend on local conditions.
Global availability and use cases for different biomass residue types
To understand the global availability of waste biomass in detail, we researched the volumes produced across the most important categories. The following graph shows our findings, with values extrapolated to a global level where data was missing. Note that we converted all values to dry biomass based on average moisture content to improve comparability.

(1) All values are dry matter per year; not including invasive species as well as non-accessible biomass like deadwood from unmanaged forests.
(2) Category includes legumes, oil crops, sugar crops and tubers. (3) Construction and Demolition Wood Waste; value includes EU, UK, US, AUS, HKG. (4) Sewage Sludge. Sources: FAO, ECF, UNEP, ourworldindata.org, academic literature
Our analysis yields a total of ~9 Gigatons of dry biomass residues produced every year. As we had to omit certain types of industrial food waste and the entire category of invasive plant species for lack of global data, this likely represents a conservative estimate. The amount of CO2 emitted from those residues depends on the decay period as well as the carbon content, which commonly varies between 35% and 55%. Assuming an average carbon content of 45%, a stable carbon yield of 50% (for pyrolysis plants optimized on biochar output), the C-to-CO2 constant of 3.67, and minor deductions for transport and processing emissions, the 9 Gt translate to at least 6 Gt of annual CO2 emissions that could theoretically be prevented.
So, what are the implications – can, and should we turn all of that biomass into biochar/biocarbon? Most certainly not; different regions will require certain shares for local needs such as the cultivation of fertile soils, animal feed, or energy production. To better understand these potential uses, let’s take a closer look at the main categories of biowaste from our chart:
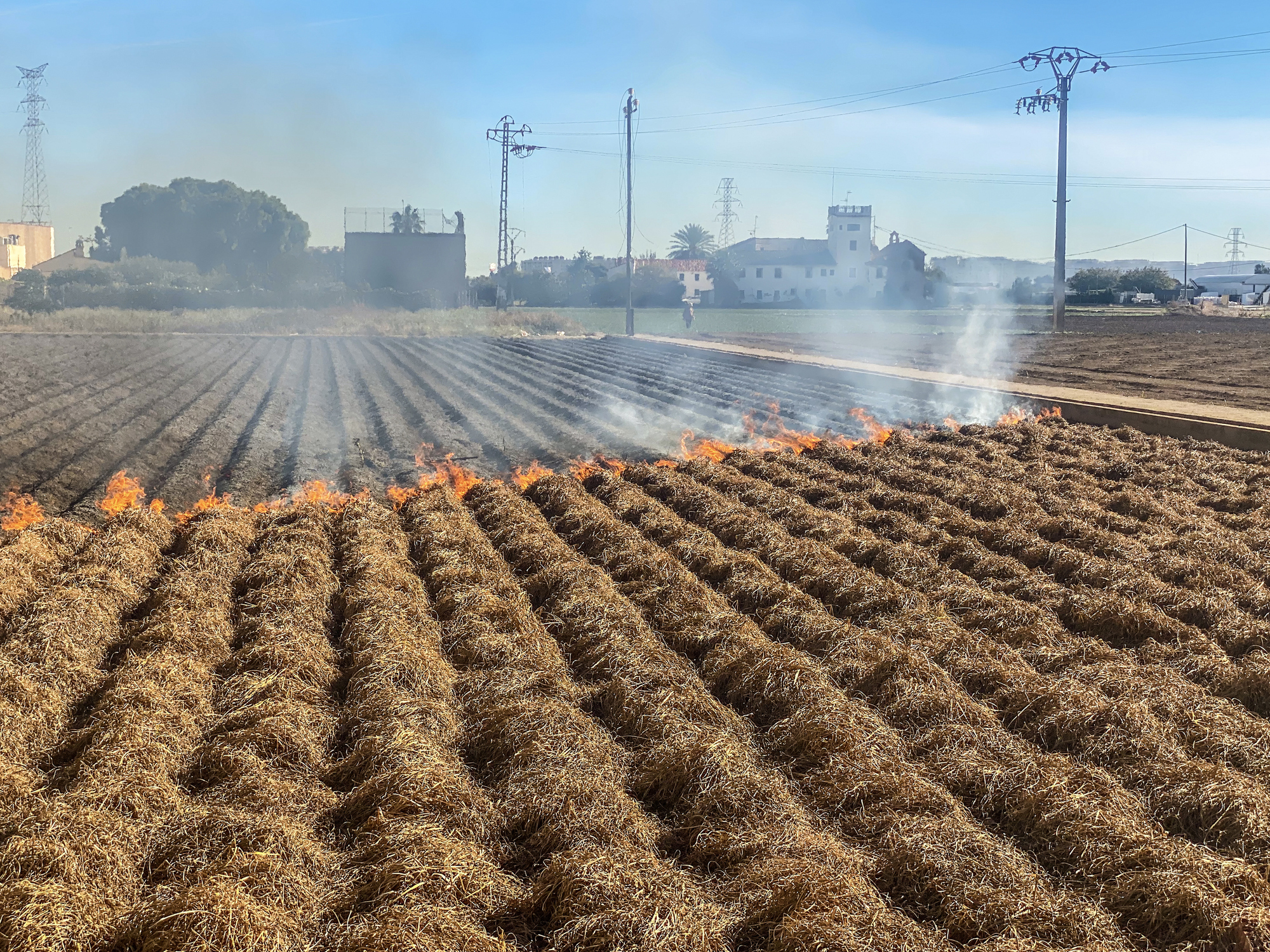
- At the top of our ranking are cereal residues from agriculture with a total volume of almost ~3 Gt of dry waste biomass produced every year. Examples are corn stover, rice straw, or wheat straw. These byproducts are frequently used as animal feed or left to decompose on the fields to support microbial activity and regenerate soils. Yet, studies show that surpluses can reach up to 50% and often are burned, directly releasing CO2. Therefore, up to 1.5 Gt of cereal residues could be available for other purposes including BCR. However, it is worth mentioning further novel use cases such as biodegradable packaging materials, which often need cellulosic biomass as a core product base. Due to the high number of solutions that are being explored, it is difficult to estimate how cereal residues will eventually be split among different sustainable purposes.
- A close follower: livestock manure, for which the FAO estimates global production at an astonishing 13 Gt wet, translating to 2.5 – 3 Gt dry. Especially in Europe, both cereal residue and manure are frequently used to produce energy in biogas plants – for example, German farmers use up to a third of manure for biogas. We conservatively estimate that about 50% of the global manure stock is in productive and sustainable use. This share may even remain constant as the efficiency of agricultural energy solutions is growing just as fast as energy demand and manure production – likely leaving more than 1 Gt of dry biomass that could be pyrolyzed every year. Integrating pyrolysis into agricultural systems could pay off in many ways, since manure and digestate are suitable BCR feedstocks, while excess heat energy from the pyrolysis process can supply greenhouses with energy, and biochar can be used as an effective soil amendment.
- The next-largest source of biowaste are forestry residues with roughly 1-1.2 Gt dry. This category includes deadwood and offcuts from managed forests, sawmill waste, and other residues arising along the forestry value chain. Typically available as wood chips, they represent one of the most popular feedstocks for BCR – but also a tricky one when it comes to additionality: users must take care not to divert critical resources from natural ecosystems or from value-added purposes like furniture production. As such considerations must be locally evaluated, it is difficult to estimate what part of forestry residues could be sustainably sourced. Regardless though, thanks to a high carbon content of 75-95% the carbon removal potential of wood-powered BCR may still get close 1 Gt of CO2 per year.
- Other agricultural residues are derived from legumes, oil crops, and cash crops like fruits, coffee, or sugar. They make up about 1 Gt of dry biowaste per year. Just like for the next-biggest category, food processing residues (450-600 Mt), an immense variety of crop parts are being discarded on a regular basis. The main challenge for BCR in this context lies in ensuring feedstock homogeneity, including consistent moisture content and chemical composition. For this reason, non-industrial food waste, such as from households, is mostly not a great fit for BCR.
- Finally, digestate, construction and demolition wood waste, and sewage sludge jointly account for about 500 Mt of annual dry biomass residues. While vastly different in terms of chemical composition and carbon content, they share a commonality: in many countries, those byproducts are strictly classified as 'waste'. In the EU, the waste tag can significantly impact their further use, as all follow-on products (e.g., biocarbon) are likewise considered waste until a certified 'end-of-waste'-process is carried out (which pyrolysis can be approved as). Furthermore, sewage sludge is a prime example of a waste biomass that contains valuable substances like phosphorus which should be extracted prior to processing. Germany has therefore passed a Sewage Sludge Ordinance governing that phosphorus from sewage sludge must remain in the cycle from 2029.

Beyond biomass-specific considerations, there are cross-cutting use cases. One example is precision fermentation in food production, which might be able to use waste biomass as a feedstock. Another is biomass sinking, a form of ocean fertilization that not only sequesters carbon but also improves oxygen levels in the deep sea. But the most prominent one is certainly combustion for energy generation. Waste biomass forms part of most national energy strategies as a reliable source of carbon-neutral peak load electricity, required until energy storage is available at scale. Moreover, biomass is often used to generated renewable heat in developing countries for lack of a better alternative. Nevertheless: for high-carbon biomass in particular, the carbon removal value per ton is usually higher than the carbon avoidance value if used purely as an energy source (a formula coined as the Aines principle and explored in multiple papers).
Modern biomass energy pathways emphasize the need to capture and geologically store the CO2 released during combustion. This process is referred to as BECCS (Biomass Energy with Carbon Capture and Storage) and, provided non-virgin resources are used as feedstocks, it combines the energetic use of a biomass with carbon removal. But even if BECCS at scale is an option, which would require large centralized facilities that combine combustion, heat-to-electricity conversion, and CCS: let’s not forget that pyrolysis also manages to extract a significant share of a biomass’ energy value (usable to produce heat or biofuels), sequesters carbon AND returns a functional material with many locally valuable applications. To conclude by quoting a very differentiated article by Lennart Joos: biomass may be too precious to simply be burned.
Conclusion: finding the best-fit purpose for biomass residues
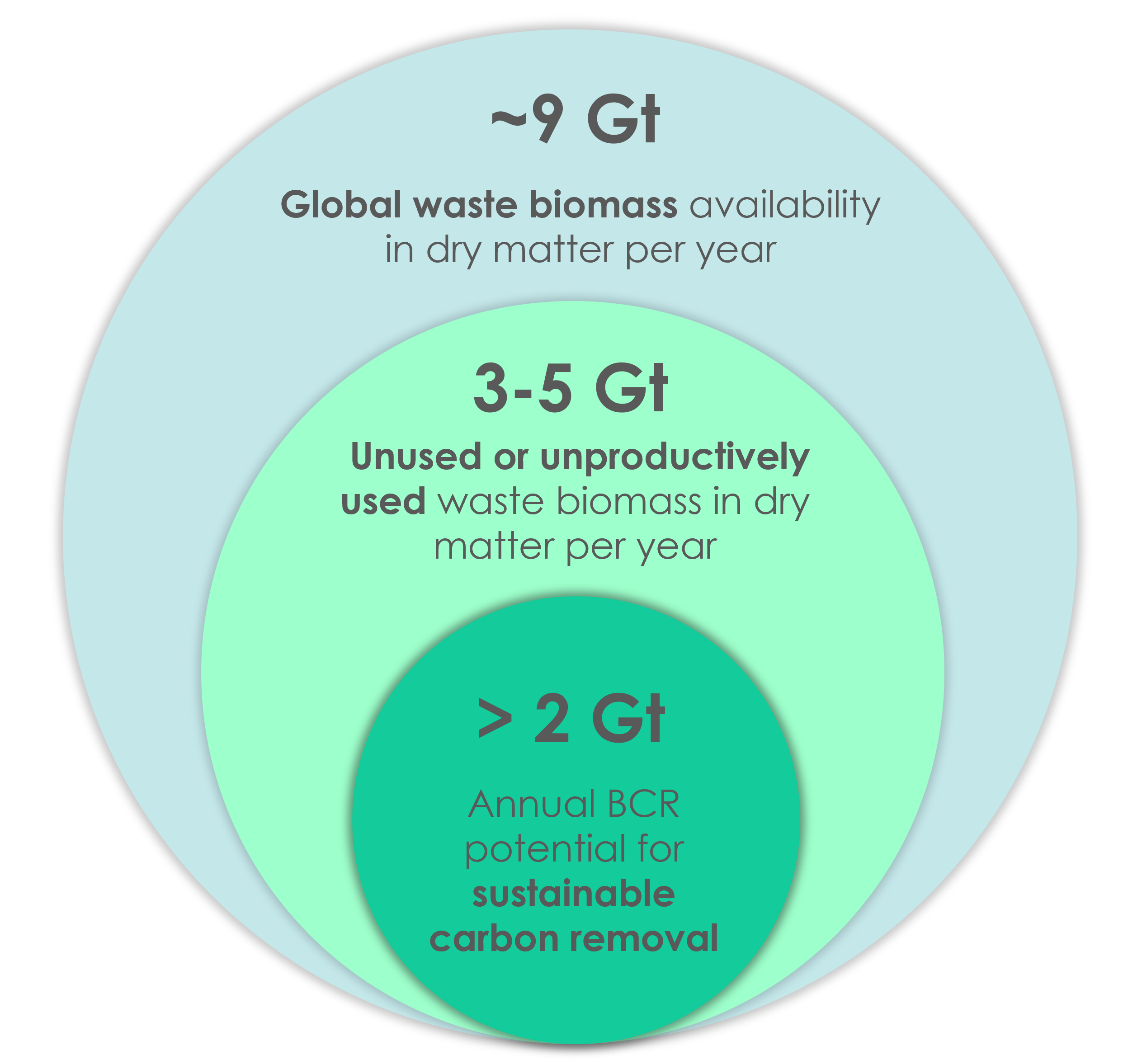
We originally approached this article using a biochar lens, aiming to find out whether enough feedstock can be sustainably sourced to power BCR at a gigaton scale. Our take: considering the effectiveness and versatility of BCR in sequestering carbon and creating functional products, a significant portion of global biomass residues should be made available for this pathway. Building upon our ~9 Gt p.a. estimate for global dry waste biomass production, we predict that 3 to 5 Gt of global waste biomass are either unused or unproductively used. This figure matches with the EBI's calculation for Europe, which yielded 0.9 to 1.2 Gt of sustainably procurable dry biomass. Revisiting the key question from our last blog article, we obtain a global carbon removal potential with BCR of at least 2 Gt of CO2 per year, well in line with the IPCC's estimate of ~2.6 Gt of CO2.
But we want to end on a broader note, asking how policymakers, businesses, and other stakeholders can identify the most beneficial way of using local biomass residues. All in all, it appears impossible to select a single best use case that will provide most value across all localities. The many possible usage forms warrant a case-by-case assessment of the environmental and social impacts of different applications in the local context. For BCR players, this means they must establish clear sustainable sourcing guidelines to advance trust for biochar products and carbon removal certificates alike. And since wood might be the trickiest feedstock to get right, producers may want to embrace additional feedstocks, which will also allow them to expand into new regions. The European Biochar Certificate’s positive list outlines what feedstock-derived biocarbons are considered suitable for specific certification levels (i.e., purposes).
As practical guidance for decision-makers, we propose the following hierarchy of questions. Note that this perspective is focused on the benefits of biomass conversion, not yet taking into account logistics, capex, lead time, and further practical considerations:
- Can the waste biomass be extracted from the local ecosystem without adversely affecting either biodiversity and ecosystem services or local communities? If yes…
- Are there any valuable substances to be extracted, such as phosphorus, that would get lost during conversion? If they can be safely extracted…
- Is there an important local need, such as animal feed or biomaterials, that cannot be served in an equally effective way with other renewable resources? If no…
- Do any regulations, such as waste directives, exclude the use of the biomass for the envisioned purpose (e.g., materials)? If no…
- Is there an impactful local application for biocarbon, e.g., in construction materials or agriculture?
- If yes: go with BCR
- If no: go with BECCS
Having explored the scalability of BCR from the supply side, in our next article we will move to the demand side: what might be a scalable end use for biocarbon that both provides a permanent carbon sink and an industry willing to pay for the material? Hint: it’s rock-solid!
Text by Mario Schmitt, Analysis by Sepanta Golchin